The basic unit of life
Charles Darwin had a conundrum. He put out the theory of natural selection in his seminal work On the Origin of Species, which was published in 1859, to explain the slow emergence and extinction of many animal and plant forms over extended periods of time. However, he came to see that the fossil record—which served as the foundation for his theory—was lacking, particularly with regard to the origin of life. When Darwin was alive, the earliest fossils that had been discovered were
sophisticated organisms from the Cambrian epoch, which dates the rocks to around 550 million years ago. Precambrian fossils were missing; where were they? These would undoubtedly offer a
connect to the source of life.
Cells are the fundamental components of life, much like atoms are the building elements of chemistry. The cell theory consists of three claims:
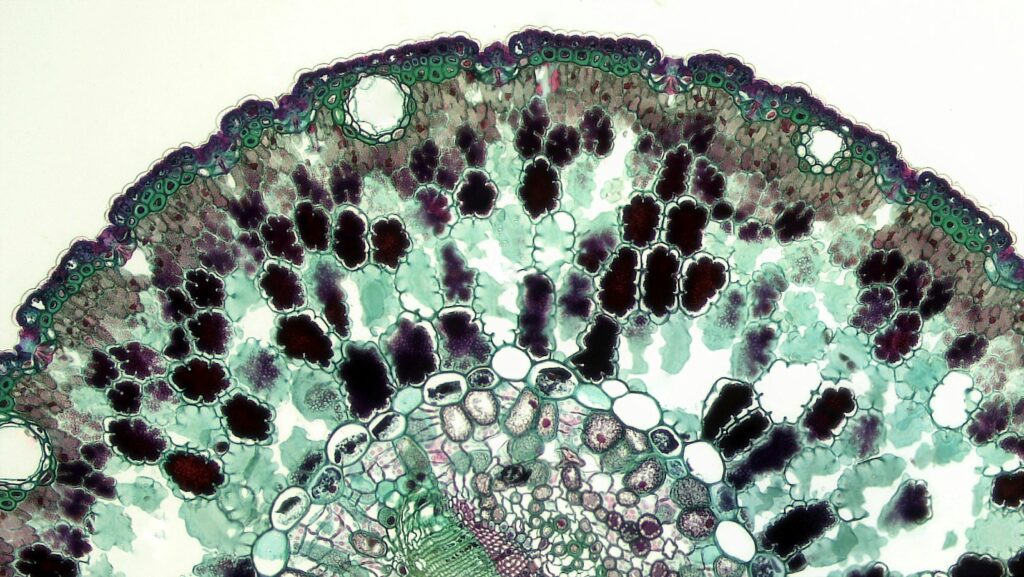
1) Life is made up of basic units called cells.
2) Every living thing is made up of cells.
3) Every cell originates from an earlier cell.
Water molecules and the small and large molecules we looked at in the previous two chapters make up cells.
At least 10,000 distinct kinds of molecules, the majority of which are found in multiple copies, are found within each cell. These chemicals are used by cells for self-replication, environmental response, and the transformation of matter and energy.
There are three major ramifications for the cell theory. First of all, it implies that researching cell biology is comparable to researching life in certain ways. The 60 trillion cells in your body are governed by comparable principles to those that underpin the activity of a single bacterial cell. It also implies that life is ongoing. The fertilized egg, which was created by the fusing of two cells—a sperm and an egg from your parents, whose cells were created from their fertilized eggs, and so on—was the source of all the cells in your body. Lastly, it indicates that the emergence of the first cells signified the beginning of life on Earth.
Potential source of cells stable bubbles:
In the lab, it is possible to obtain isolation from the surrounding environment by using aggregates comprised of molecules created during prebiotic synthesis studies.
These aggregates, known as protobionts, are incapable of procreating yet are able to preserve chemical conditions inside them that are distinct from their external environs. Under a microscope, they have the appearance of tiny cells.
Russian scientist Alexander Oparin combined a big protein and a polysaccharide in a solution in the 1920s. Bubbles appeared in this mixture when he stirred it. He might apply this to other polymers as well. The interiors of these bubbles had much higher quantities of the macromolecules than their surroundings. They also had some control over what left them and passed the border into the environment, and they catalyzed chemical reactions. Put otherwise, they were protobionts. Subsequent studies revealed that when lipids are combined with water, they spontaneously organize into droplets that are encircled by a bilayer.
Cell size is constrained by the ratio of surface area to volume:
The majority of cells are small. Cells can have volumes ranging from 1 to 1,000 µm3.
Certain bird eggs are definitely the exception, and individual cells of various bacteria and algae species are big enough to be seen with the unaided eye. Additionally, nerve cells, or neurons, have a volume that falls within the range of “normal” cells, but they frequently contain minute projections that can reach several meters, which allow them to transmit impulses throughout a huge animal. However, most cells are very small. This is due to the fact that when an item gets bigger, its surface area-to-volume ratio (SA/V) changes.
As a cell increases in volume, its surface area likewise rises, although not to the same level. There are two major biological implications to this phenomenon:
1) The quantity of chemical activity a cell does in a given amount of time is determined by its volume.
2) The surface area of a cell determines the amount of substances the cell can take in from the outside environment and the amount of waste products it may emit to the environment.
A live cell’s rate of waste production and resource consumption rise more quickly as it gets bigger than its surface area. This clarifies the requirement for numerous little cells to make up huge organisms: To sustain a high surface area-to-volume ratio, cells have a limited volume.
A multicellular organism can perform the vast array of tasks necessary for survival because of the immense surface area that each of its many tiny cells represents. Food, oxygen, and waste products are transported to and from the little cells, which are located far from the organism’s exterior, by use of special structures.
Why Do Cells Have Such Small Sizes?
An object’s volume expands faster than its surface area as it gets bigger. Large organisms must be able to maintain a high surface area-to-volume ratio because cells need this to function.